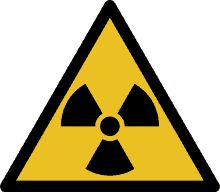
Radiation dosimetry is the measurement, calculation and assessment of the absorbed doses and assigning those doses to individuals. It is the science and practice that attempts to quantitatively relate specific measures made in a radiation field to chemical and/or biological changes that the radiation would produce in a target.
Since there are two types of radiation exposure, external and internal exposure, dosimetry may be also categorized as:
- External Dosimetry. External exposure is radiation that comes from outside our body and interacts with us. In this case, we analyze predominantly exposure from gamma rays and beta particles, since alpha particles, in general, constitute no external exposure hazard because the particles generally do not pass through skin. Since photons and beta interact through electromagnetic forces and neutrons interact through nuclear forces, their detection methods and dosimetry are substantially different. The source of radiation can be, for example, a piece of equipment that produces the radiation like a container with a radioactive materials, or like an x-ray machine. External dosimetry is based on measurements with a dosimeter, or inferred from measurements made by other radiological protection instruments.
HPGe detector with LN2 cryostat, which can be used in whole-body counters. Source: canberra.com Internal Dosimetry. If the source of radiation is inside our body, we say, it is internal exposure. The intake of radioactive material can occur through various pathways such as ingestion of radioactive contamination in food or liquids. Protection from internal exposure is more complicated. Most radionuclides will give you much more radiation dose if they can somehow enter your body, than they would if they remained outside. Internal dosimetry assessment relies on a variety of monitoring, bio-assay or radiation imaging techniques.
Personal Dosimetry
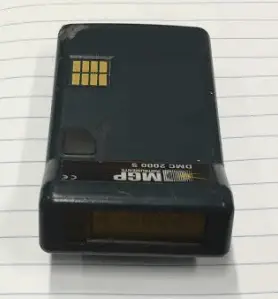
Personal dosimetry is a key part of radiation dosimetry. Personal dosimetry is used primarily (but not exclusively) to determine doses to individuals who are exposed to radiation related to their work activities. These doses are usually measured by devices known as dosimeters. Dosimeters usually record a dose, which is the absorbed radiation energy measured in grays (Gy) or the equivalent dose measured in sieverts (Sv). A personal dosimeter is dosimeter, that is worn at the surface of the body by the person being monitored, and it records of the radiation dose received. Personal dosimetry techniques vary and depend partly on whether the source of radiation is outside the body (external) or taken into the body (internal). Personal dosimeters are used to measure external radiation exposures. Internal exposures are typically monitored by measuring the presence of nuclear substances in the body, or by measuring nuclear substances excreted by the body.
Commercially available dosimeters range from low-cost, passive devices that store personnel dose information for later readout, to more expensive, battery operated devices that display immediate dose and dose rate information (typically an electronic personal dosimeter). Readout method, dose measurement range, size, weight, and price are important selection factors.
There are two kinds of dosimeters:
- Passive Dosimeters. Commonly used passive dosimeters are the Thermo Luminescent Dosimeter (TLD) and the film badge. A passive dosimeter produces a radiation-induced signal, which is stored in the device. The dosimeter is then processed and the output is analyzed.
- Active Dosimeters. To get a real time value of your exposure you can instead use an active dosimeter, typically an electronic personal dosimeter (EPD). An active dosimeter produces a radiation-induced signal and displays a direct reading of the detected dose or dose rate in real time.
The passive and the active dosimeters are often used together to complement each other. To estimate effective doses, dosimeters must be worn on a position of the body representative of its exposure, typically between the waist and the neck, on the front of the torso, facing the radioactive source. Dosimeters are usually worn on the outside of clothing, around the chest or torso to represent dose to the “whole body”. Dosimeters may also be worn on the extremities or near the eye to measure equivalent dose to these tissues.
The personal dosimeters in use today are not absolute instruments, but reference instruments. That means , they must be periodically calibrated. When a reference dosimeter is calibrated, a calibration factor can be determined. This calibration factor relates the exposure quantity to the reported dose. Validity of the calibration is demonstrated by maintaining traceability of the source used to calibrate the dosimeter. The traceability is achieved by comparison of the source with a “primary standard” at a reference calibration centre. In monitoring of individuals, the values of these operational quantities are taken as a sufficiently precise assessment of effective dose and skin dose, respectively, in particular, if their values are below the protection limits.
Medical Dosimetry
Medical dosimetry is the calculation of absorbed dose and optimization of dose delivery in medical examinations and treatments. In general, radiation exposures from medical diagnostic examinations are low (especially in diagnostic uses). Doses may be also high (only for therapeutic uses), but in each case, they must be always justified by the benefits of accurate diagnosis of possible disease conditions or by benefits of accurate treatment. These doses include contributions from medical and dental diagnostic radiology (diagnostic X-rays), clinical nuclear medicine and radiation therapy. Medical dosimetry is often performed by a professional health physicist with specialized training in that field. In order to plan the delivery of radiation therapy, the radiation produced by the sources is usually characterized with percentage depth dose curves and dose profiles measured by a medical physicist.
The medical use of ionizing radiation remains a rapidly changing field. In any case, usefulness of ionizing radiation must be balanced with its hazards. Nowadays a compromise was found and most of uses of radiation are optimized. Today it is almost unbelievable that x-rays was, at one time, used to find the right pair of shoes (i.e. shoe-fitting fluoroscopy). Measurements made in recent years indicate that the doses to the feet were in the range 0.07 – 0.14 Gy for a 20 second exposure. This practice was halted when the risks of ionizing radiation were better understood.
See also: Medical Exposures
Environmental Dosimetry
Environmental dosimetry is used where it is likely that the environment will generate a significant radiation dose. As was written, radiation is all around us. In, around, and above the world we live in. It is a natural energy force that surrounds us. It is a part of our natural world that has been here since the birth of our planet. All living creatures, from the beginning of time, have been, and are still being, exposed to ionizing radiation. Ionizing radiation is generated through nuclear reactions, nuclear decay, by very high temperature, or via acceleration of charged particles in electromagnetic fields.
In general, there are two broad categories of radiation sources in the environment:
- Natural Background Radiation. Natural background radiation includes radiation produced by the Sun, lightnings, primordial radioisotopes or supernova explosions etc.
- Man-Made Sources of Radiation. Man-made sources include medical uses of radiation, residues from nuclear tests, industrial uses of radiation etc.
An example of environment dosimetry is radon monitoring. Radon is a radioactive gas generated by the decay of uranium, which is present in varying amounts in the earth’s crust. It is important to note that radon is a noble gas, whereas all its decay products are metals. The main mechanism for the entry of radon into the atmosphere is diffusion through the soil. Certain geographic areas, due to the underlying geology, continually generate radon which permeates its way to the earth’s surface. In some cases the dose can be significant in buildings where the gas can accumulate. Locations with higher radon background are well mapped in each country. In the open air, it ranges from 1 to 100 Bq/m3, even less (0.1 Bq/m3) above the ocean. In caves or aerated mines, or ill-aerated houses, its concentration climbs to 20–2,000 Bq/m3. In the outdoor atmosphere, there is also some advection caused by wind and changes in barometric pressure. A number of specialised dosimetry techniques are used to evaluate the dose that a building’s occupants may receive.
Radiation Dose Measuring and Monitoring
In previous chapters, we described the equivalent dose and the effective dose. But these doses are not directly measurable. For this purpose, the ICRP has introduced and defined a set of operational quantities, which can be measured and which are intended to provide a reasonable estimate for the protection quantities. These quantities aim to provide a conservative estimate for the value of the protection quantities related to an exposure avoiding both underestimation and too much overestimation.
Numerical links between these quantities is represented by conversion coefficients, which are defined for a reference person. It is very important that an internationally agreed set of conversion coefficients is available for general use in radiological protection practice for occupational exposures and exposures of the public. For the calculation of conversion coefficients for external exposure, computational phantoms are used for dose assessment in various radiation fields. For the calculation of dose coefficients from intakes of radionuclides, biokinetic models for radionuclides, reference physiological data, and computational phantoms are used.
A set of evaluated data of conversion coefficients for protection, and operational quantities for external exposure to mono-energetic photon, neutron, and electron radiation under specific irradiation conditions is published in reports (ICRP, 1996b, ICRU, 1997).
In general, the ICRP defines operational quantities for area and individual monitoring of external exposures. The operational quantities for area monitoring are:
- Ambient dose equivalent, H*(10). The ambient dose equivalent is an operational quantity for area monitoring of strongly penetrating radiation.
- Directional dose equivalent, H’ (d,Ω). The directional dose equivalent is an operational quantity for area monitoring of weakly penetrating radiation.
The operational quantities for individual monitoring are:
- Personal dose equivalent, Hp(0.07). The Hp(0.07) dose equivalent is an operational quantity for individual monitoring for the assessment of the dose to the skin and to the hands and feet.
- Personal dose equivalent, Hp(10). The Hp(10) dose equivalent is an operational quantity for individual monitoring for the assessment of effective dose.
Special Reference: ICRP, 2007. The 2007 Recommendations of the International Commission on Radiological Protection. ICRP Publication 103. Ann. ICRP 37 (2-4).
Dose Limits
See also: Dose Limits
Dose limits are split into two groups, the public, and occupationally exposed workers. According to ICRP, occupational exposure refers to all exposure incurred by workers in the course of their work, with the exception of
- excluded exposures and exposures from exempt activities involving radiation or exempt sources
- any medical exposure
- the normal local natural background radiation.
The following table summarizes dose limits for occupationally exposed workers and for the public:
Source of data: ICRP, 2007. The 2007 Recommendations of the International Commission on Radiological Protection. ICRP Publication 103. Ann. ICRP 37 (2-4).
According to the recommendation of the ICRP in its statement on tissue reactions of 21. April 2011, the equivalent dose limit for the lens of the eye for occupational exposure in planned exposure situations was reduced from 150 mSv/year to 20 mSv/year, averaged over defined periods of 5 years, with no annual dose in a single year exceeding 50 mSv.
Limits on effective dose are for the sum of the relevant effective doses from external exposure in the specified time period and the committed effective dose from intakes of radionuclides in the same period. For adults, the committed effective dose is computed for a 50-year period after intake, whereas for children it is computed for the period up to age 70 years. The effective whole-body dose limit of 20 mSv is an average value over five years. The real limit is 100 mSv in 5 years, with not more than 50 mSv in any one year.
Sievert – Unit of Equivalent Dose
In radiation protection, the sievert is a derived unit of equivalent dose and effective dose. The sievert represents the equivalent biological effect of the deposit of a joule of gamma rays energy in a kilogram of human tissue. Unit of sievert is of importance in radiation protection and was named after the Swedish scientist Rolf Sievert, who did a lot of the early work on radiation dosimetry in radiation therapy.
As was written, the sievert is used for radiation dose quantities such as equivalent dose and effective dose. Equivalent dose (symbol HT) is a dose quantity calculated for individual organs (index T – tissue). Equivalent dose is based on the absorbed dose to an organ, adjusted to account for the effectiveness of the type of radiation. Equivalent dose is given the symbol HT. The SI unit of HT is the sievert (Sv) or but rem (roentgen equivalent man) is still commonly used (1 Sv = 100 rem).
Examples of Doses in Sieverts
We must note that radiation is all around us. In, around, and above the world we live in. It is a natural energy force that surrounds us. It is a part of our natural world that has been here since the birth of our planet. In the following points we try to express enormous ranges of radiation exposure, which can be obtained from various sources.
- 0.05 µSv – Sleeping next to someone
- 0.09 µSv – Living within 30 miles of a nuclear power plant for a year
- 0.1 µSv – Eating one banana
- 0.3 µSv – Living within 50 miles of a coal power plant for a year
- 10 µSv – Average daily dose received from natural background
- 20 µSv – Chest X-ray
- 40 µSv – A 5-hour airplane flight
- 600 µSv – mammogram
- 1 000 µSv – Dose limit for individual members of the public, total effective dose per annum
- 3 650 µSv – Average yearly dose received from natural background
- 5 800 µSv – Chest CT scan
- 10 000 µSv – Average yearly dose received from natural background in Ramsar, Iran
- 20 000 µSv – single full-body CT scan
- 175 000 µSv – Annual dose from natural radiation on a monazite beach near Guarapari, Brazil.
- 5 000 000 µSv – Dose that kills a human with a 50% risk within 30 days (LD50/30), if the dose is received over a very short duration.
We hope, this article, Radiation Dosimetry, helps you. If so, give us a like in the sidebar. Main purpose of this website is to help the public to learn some interesting and important information about radiation and dosimeters.